One crucial aspect of any research and development is to record and analyze data, which then can be used for quantifying performance, debugging strange behavior, or guide us in our decision making. We have been trying to improve the way that this is done to help all of you researcher out there with their work, so this blog post will explain an alternative, better, method to record whatever is happening on the Crazyflie in real-time.
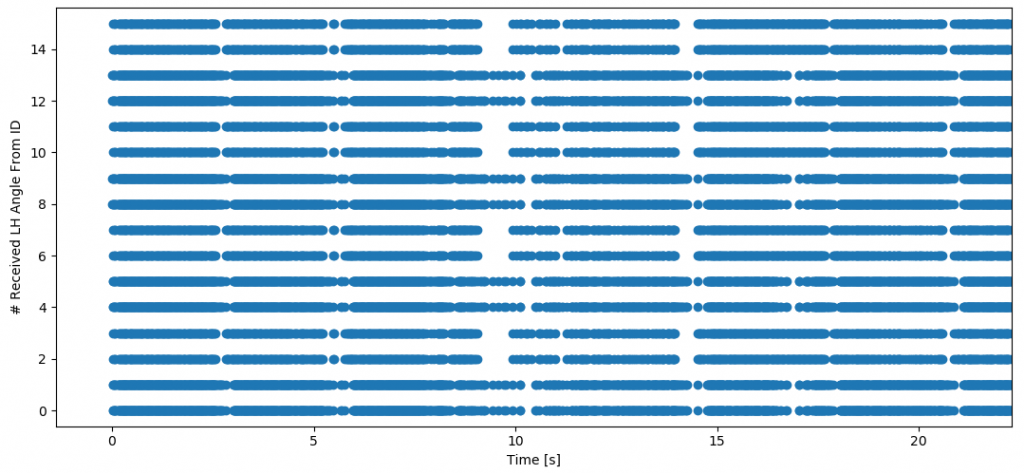
Existing Logging Approaches
So far there have been two principle ways of recording data with the Crazyflie:
- Logging: In the firmware, one can define global variables that can be streamed out at a fixed frequency, using logging configurations. Variables have a name and data type, and the list of all available variables can be queried from the firmware.
- Debug Prints: It is possible to add DEBUG_PRINT(…) in any place that contains a string and possibly some variables. These are asynchronous, but not timestamped, so they are mostly useful to notify the user of some status change.
Both approaches have multiple backends. The logging variables can be streamed over USB, via the Crazyradio, or on a Micro-SD-card using a specialized deck. When using the radio or USB, the frequency is limited to 100 Hz and only a few variables can be streamed at this speed due to bandwidth limitations. The Micro-SD-card deck, on the other hand, allows to log a lot more logging variables at speeds up to 1 kHz, making it possible to access high-speed sensor information, e.g., the IMU data. The Debug Prints can be used over the radio or USB, or with a SEGGER J-Link and a Debug Adapter, over J-Link Real-Time Transfer (RTT). The former is very bandwidth limited, while the latter requires a physical wire connection, making it impossible to use while flying.
New Approach: Event-Based Logging
Event-based logging combines the advantages of the existing approaches and offers a third alternative to record data. Similar to debug prints, a user can trigger an event anywhere in the code and include some mandatory variables that describe this event (so-called payload). Similar to logging, these events are timestamped and have fewer bandwidth limitations. Currently, there is just one backend for the Micro-SD-card deck, but it would be possible to add support for radio and USB as well.
One of our first test cases of the event-based logging is to analyze the data we get from the Lighthouse deck. Here, we trigger an event whenever we received a raw sweep. This allows us to visually see the interference that happens between two LH2 basestations. We also use the event-based logging for time synchronization of the Crazyflie and a motion capture system: when we enable the IR LEDs on the active marker deck, we record an event that contains the Crazyflie timestamp. On the PC side, we record a PC timestamp when the motion capture system first detects the IR LEDs. Clock drifts can be computed by using the same logging mechanism when the IR LEDs are turned off.
Adding event-based logging had some other good side effects as well: the logging is now generally much faster, there is more user feedback about the correct buffer size usage, and the binary files are smaller. More details are in the documentation.
Future Work
We are working on adding event triggers to the state estimator. This will enable us to record all the sensor information during real flights with different positioning systems, so that we can tune and improve the Kalman state estimator. It will also be interesting to add support for events in CRTP, so that it can be used over USB and radio.